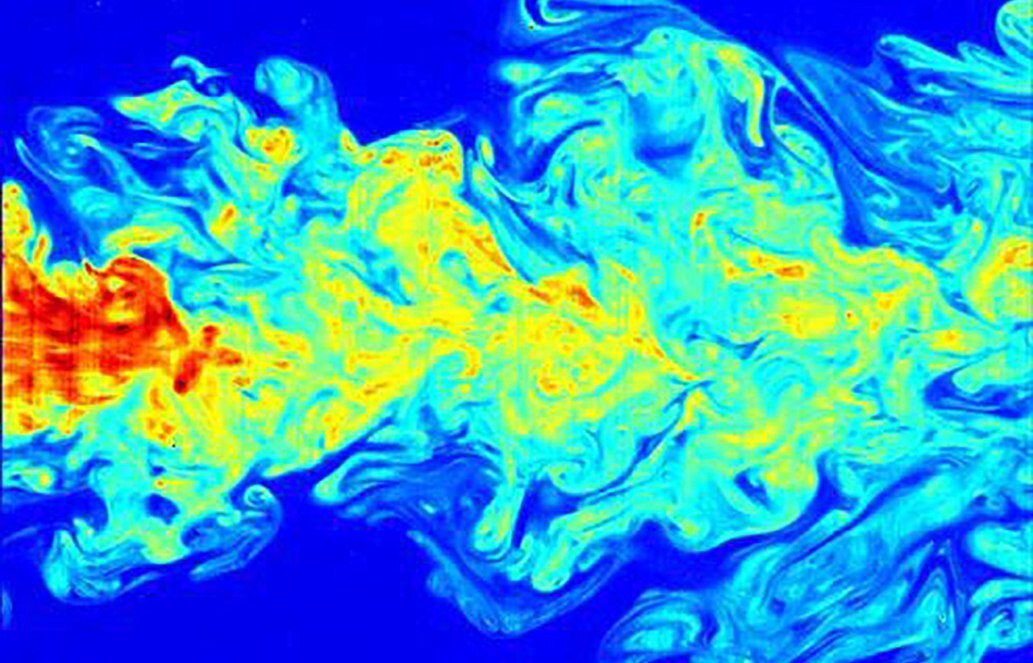
New to turbulent flow?
- Nortek Wiki
1. The concept of turbulent flow
Although you might be new to the concept of turbulent flow, if you have ever flown in an airplane, you have certainly experienced turbulence first hand! So even if our main concern is water, let us try to think about how we perceive turbulent air. Even though an airplane moves through a medium that is invisible and not very dense, the plane almost never flies smoothly, right? There are always bumps and jolts along the way on flights.
This is because the air the airplane flies through is not moving with uniform speed and does not have a uniform density. Rather, some parts of the air are moving faster than others, and sometimes in different directions, while some parts are less dense than others. If all parts of the air were flowing uniformly, and they all had the same density, then we would call this laminar flow. When this is not the case, we call it turbulent flow.
When an airplane encounters a portion of air that is moving in a different way (or has a different density) than the air around it, the airplane experiences a bump. If the variations in the air speed (or density) are small, you feel a small bump. If the variations are large, you feel a correspondingly large bump.
Furthermore, if the variations are large, but the volume of air is small – such that it spans only a small portion of the airplane – then you only feel a small bump (if you feel any bump at all). But if the volume covers a large portion of the airplane, then you feel a large bump. So, as you have likely deduced, your sense of how big the bump is depends on the characteristics of the air that the plane flies through.
1.1 Turbulent flow in the ocean
Water in the oceans can also exhibit turbulent characteristics. Just as turbulent flow is an undesirable phenomenon for an airplane, it can also cause problems in the ocean. For example, when an underwater structure is exposed to turbulent flows, it experiences more stress than if the flow was laminar, and that can lead to structural fatigue and maybe even premature failure.
But turbulence also has some positive aspects. For instance, without turbulent flows, mixing processes between different water masses would be very slow, and the same is true with energy exchange between the ocean and the atmosphere. Modelling this process correctly has direct implications for our understanding of climate change.
2. How are turbulent flows measured?
In nature, and especially underwater, turbulent flows vary widely, both with distance and with time.
A simple illustration is given by soap bubbles. When exposed to a gentle breeze, a small soap bubble will move through the air in a turbulent manner, but will retain its spherical form. A very large soap bubble, on the other hand, will also move through the same air in a turbulent manner; however, it will conform to the turbulence patterns in the flow as it seeks to maintain a minimal surface area, being deformed by the varying pressure and velocity fluctuations in the air. Even though both bubbles are exposed to the same air mass, the spatial scales impact each bubble differently.
Although not as easily observed, the temporal scale also impacts how the bubbles behave. If the air flows faster, the small bubble’s movement will be more chaotic, while the deformation of the large bubble will be greater.
The same principle exists underwater. But unlike in air, the flows are harder to detect without specialized equipment. This is because light travels only short distances underwater, as organic and inorganic material suspended in the water blocks and scatters it, making the use of light effective only at very short ranges (typically a few meters, even in clear ocean water).
Sound, on the other hand, can travel great distances with minimal signal loss, making it an excellent choice as a carrier signal for observations underwater. Acoustic Doppler devices use sound waves and the Doppler Effect to measure velocity fluctuations underwater. This makes them powerful tools for capturing the chaotic behavior of turbulent flows.
Because underwater turbulent flows exist in both large and small scales, acoustic Doppler systems also come in large- and small-scale versions. Systems designed for large-scale measurements have divergent acoustic beams that send sound waves outward, away from the instrument. Systems designed for small scales tend to have converging acoustic beams that focus their sound waves inwardly towards a small sampling volume.
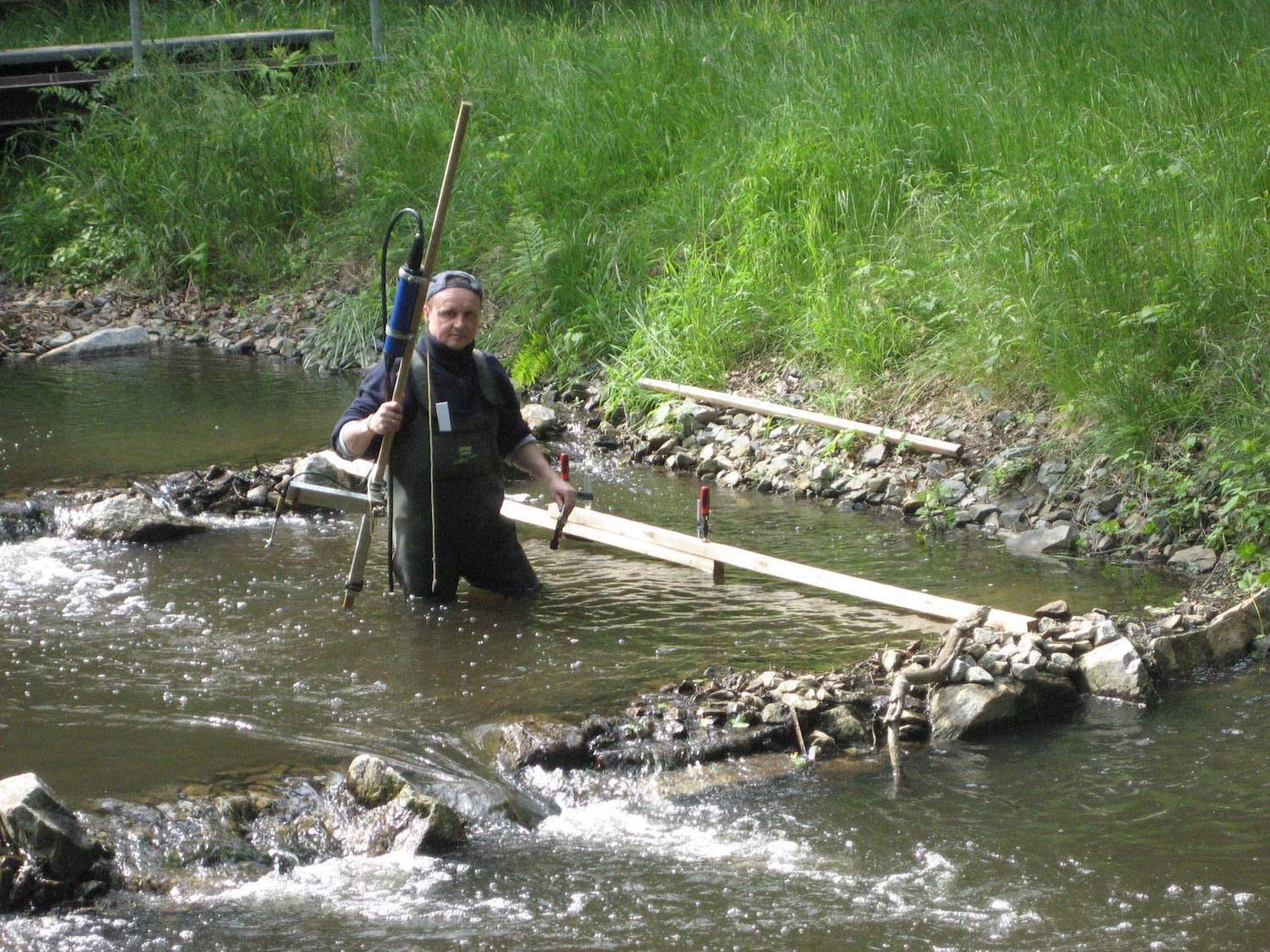
2.1 What’s needed to make high-quality measurements?
The different turbulence scales in nature are not independent, and normally coexist in the same flow. Looking down on a river from a bridge you may have observed this: as the water flows past the bridge piles, big eddies (whirls) are created, with smaller eddies spinning off the bigger eddies, and lesser eddies spinning off from these. This cascading process exists because the inertial forces in the fluid work to dampen the flow variations, while the water speed works to create turbulence.
In order to measure the rapid velocity fluctuations within these eddies, acoustic Doppler systems must sample quickly enough to resolve the variations. The importance of fast sampling cannot be overstated. Without sufficient individual (and independent) measurements of the flow, the resulting data will not contain enough detail to describe the phenomenon. Imagine if you were looking at the river only once every few minutes, for example – would you be able to describe in detail where each whirling eddy was located, their sizes, and relative spinning rates? Probably not.
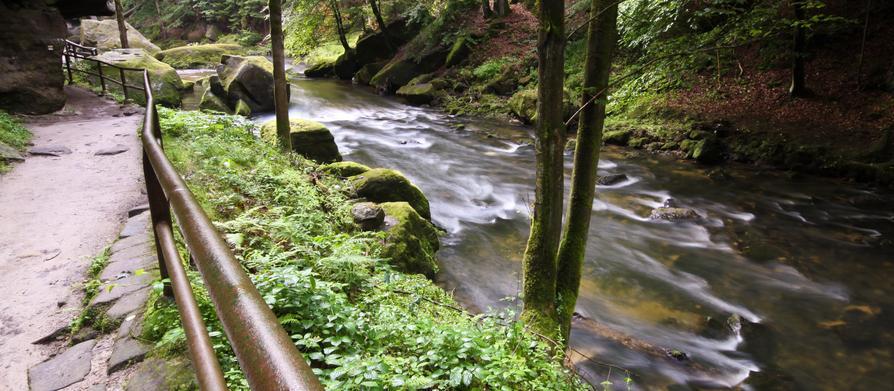
In addition to fast sampling rates, the scale of your measurement volume must match the scale of the turbulence that you are studying. The smaller the turbulence spatial scale, the smaller the measurement volume must be in order to properly describe it. If the scales do not match, you will only capture a portion of the energy within the flow.
The ability to describe a turbulent flow in three dimensions (two horizontal directions and one vertical) is also important. The world’s oceans are much wider than they are deep – even though they are very deep in places – so most fluid motion in the ocean is in the horizontal plane.
However, the vertical velocity component of turbulent flows is a key contributor to the mixing of different water masses and the exchange of energy, nutrients and particulates. Therefore, the ability to directly measure the rapid velocity fluctuations in the vertical direction is critical for high-quality turbulent-flow measurements.
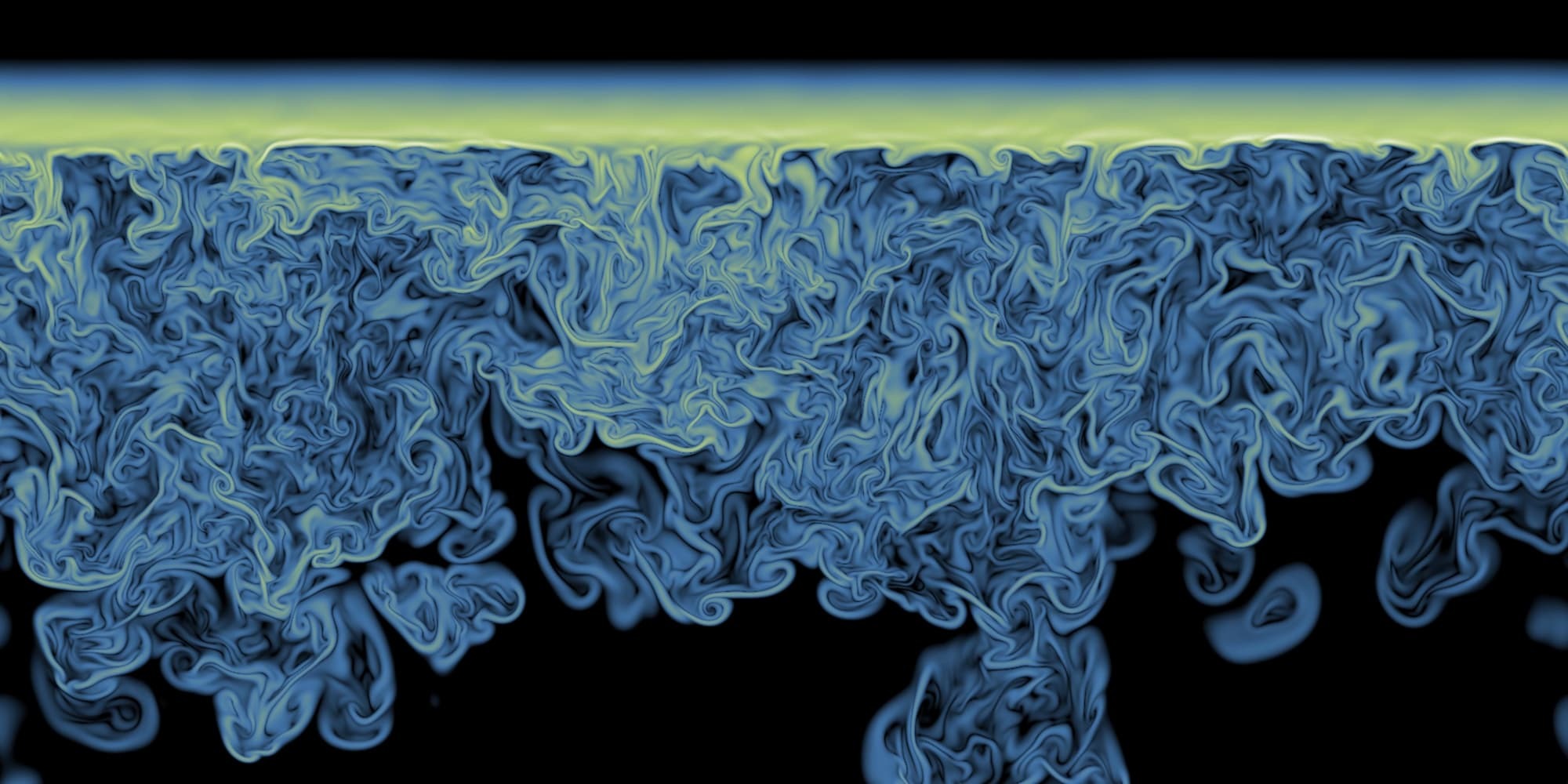
2.2 Equipment for turbulent-flow measurement
For small-scale turbulence phenomena, acoustic Doppler systems that have wide use include velocimeters such as the Vector.
For large-scale turbulence, Nortek’s Signature 1000 and Signature 500 Acoustic Doppler Current Profilers (ADCPs) offer unprecedented capabilities to characterize turbulent flows.
Velocimeters are more suited for small-scale flows, because they have convergent beams that focus the measuring point in a small volume, and also because they can sample very quickly (up to 200 Hz). ADCPs, on the other hand, have divergent beams that focus on separate parts of the water column and have limitations in terms of the minimum detectable spatial scale. Additionally, ADCPs have inherently slower sampling rates than velocimeters and cannot detect fast variations (even though Nortek’s Signature 1000 ADCP is capable of sampling up to 8 Hz on five beams – faster than any other ADCP in the world). In either case, Nortek provides users with powerful systems for measurement of turbulent flows.
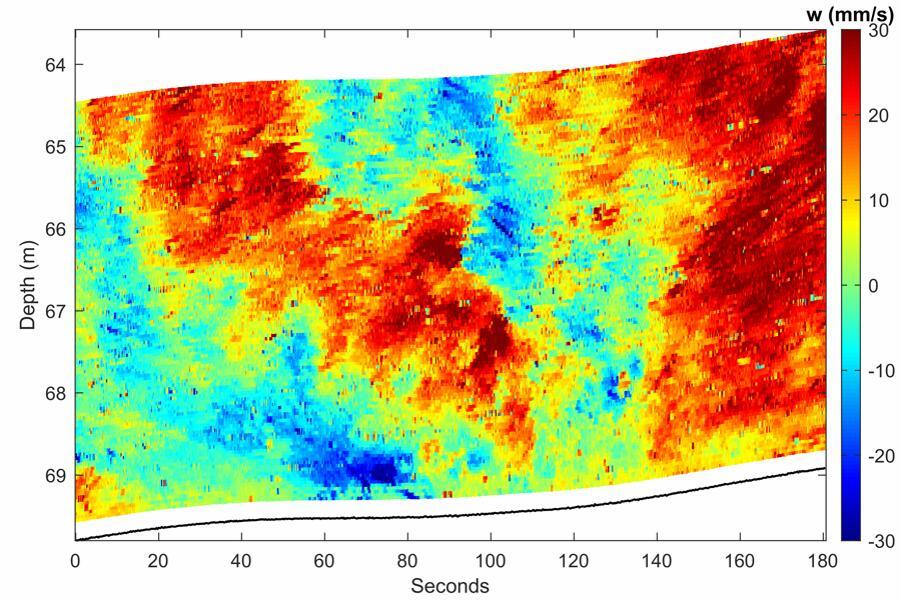